The Next Quantum Leap
Brown researchers prepare for breakthroughs in quantum science.
Brown researchers prepare for breakthroughs in quantum science.
ZOOM FAR ENOUGH IN TO ANY OBJECT—past cells, molecules, and even atoms—and you’ll eventually enter the strange world of quantum physics. At this minute scale, the universe is governed by truly bizarre rules, where particles and waves of energy are one and the same, and seemingly impossible feats like teleportation are the norm. It’s a weird and enigmatic place, yet it may be the key to creating new materials and devices that could utterly change our world— from ultra-thin superconductors to tiny computers that mimic the human brain—if, that is, we can unravel its secrets.
New research at Brown is leading the way toward that future, with dozens of scientists working on the quantum puzzle from multiple angles. Together, they’re developing new ways to both understand and harness the quantum world’s strange traits, starting with its most fundamental properties.
“Right now, we’re at the basics of how we prove this stuff. Can we actually figure out what quantum interactions really are, and can we control them to make a useful device?” said Vesna Mitrovic, a condensed matter physicist at Brown. Her lab is starting to answer those questions by focusing on one of the biggest conundrums of quantum science: measuring the basic states of particles like electrons, which act as the quantum glue that binds together every molecule.
It’s a tall order. Recording the energy level of a particle at any given moment ultimately changes that energy, so by necessity Mitrovic takes an oblique approach. She’s developed techniques that instead look at the spin of the atom’s nucleus, which is influenced by the movement of electrons around it. Using this method, she’s starting to probe the behavior of electrons on a quantum level, providing data that can inform her colleagues’ work.
Other Brown researchers, like chemistry and physics professor Brenda Rubenstein, take a different approach to a similar problem. Instead of measuring quantum behavior directly, Rubenstein’s computer models predict how subatomic particles will behave in new materials, making it possible to test their properties before they’re created. Her work could eventually provide a way to engineer quantum materials from the ground up, leading to new batteries, solar panels, and other electronic components that are more efficient than anything currently available.
“I’m really motivated by finding and developing these materials so we can make those kinds of devices in the future,” she said. “It’s incredibly exciting to me that we can use quantum phenomena and quantum theories to solve problems nobody has been able to solve before.”
Some of Rubenstein’s colleagues at Brown are already moving towards that future on a practical level, applying their quantum knowledge to create and test new materials.
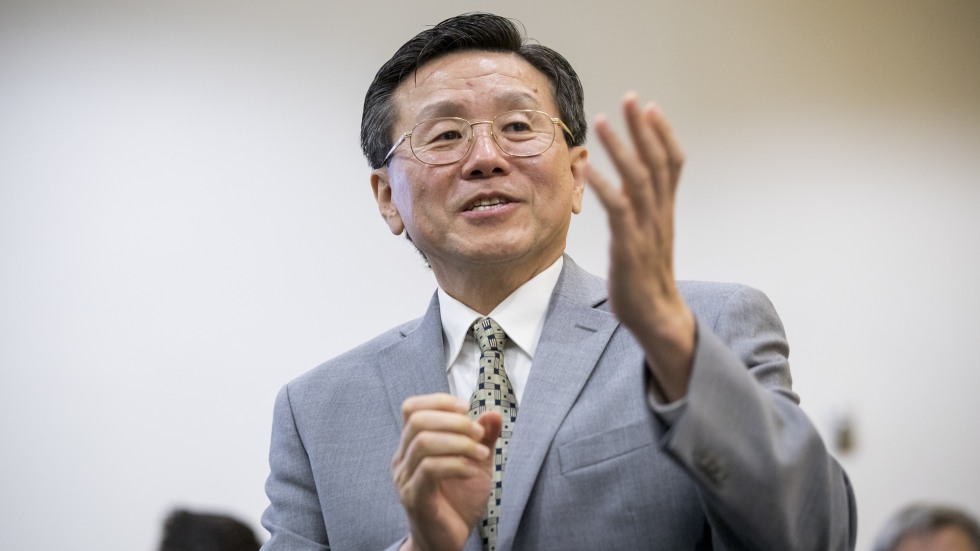
Gang Xiao, professor of physics and engineering
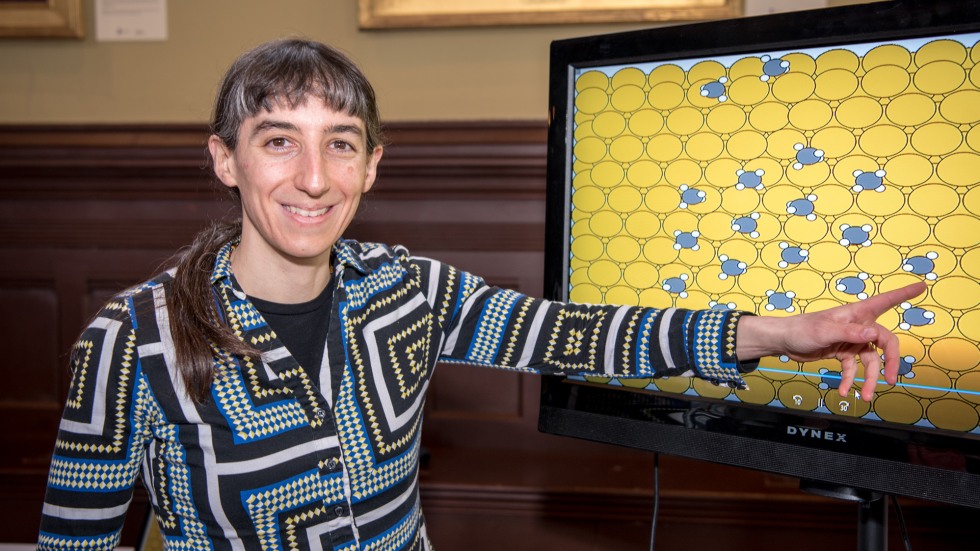
Brenda Rubenstein, associate professor of chemistry and physics, points to a simulation of methane absorbing to a gold electrode in a quantum computer
Physics department interim chair Gang Xiao, professor of physics and engineering, is building devices that generate skyrmions—disk-like magnetic swirls in two-dimensional metallic films—that can change their polarity, motion, and size when exposed to a magnetic field or an electric current. Xiao is already using skyrmions to generate truly random numbers, which might be useful in cybersecurity and encryption, yet he has his sights set higher. Arrays and networks of skyrmions, he said, could form the basis for tiny yet incredibly efficient computers.
Like traditional silicon computer processors, these devices would still be based on ones and zeros (or, in the case of skyrmions, a larger or smaller size), but the way information flows through the processor would be vastly different. When a skyrmion oscillates its size, it changes the state of its neighbors, creating a cascading effect that is eerily similar to the way neural circuits in the brain function—meaning that this sort of device could make huge strides in computing power while requiring only a small fraction of the energy required by existing computers.
“ If you built a silicon computer that mimics the human brain, you’d need a nuclear power plant to run it. But humans only need about ten watts of energy to power our brains. ”
On the surface, the work of scientists like these may seem abstract and disconnected, but Brown’s community of quantum scientists are all moving towards a common goal. When taken together, said Rubenstein, each baby step that she and her colleagues make toward understanding the quantum world brings them closer to developing revolutionary new technologies. Eventually, she’s confident that this research will lead to machines that process vast amounts of data quickly and efficiently, enabling breakthroughs in medicine, chemistry, communications, and many other fields.
“We’re still in the early stages,” said Rubenstein. “We’ve got a lot of the individual pieces figured out, but the next step is, how do we put all those things together? If we can do that, quantum science has the potential to transform people’s lives.”